Unraveling the Best Nitric Oxide Boosters
What are the best nitric oxide boosters? The quest for optimal health often leads us to natural solutions like Arginine and beets, both known for their ability to boost nitric oxide levels. In this comprehensive exploration, we delve into the science behind these two powerhouses, evaluating their effectiveness and understanding the role of nitric oxide in the human body and to understand what are the best nitric oxide boosters.
The Nitric Oxide Story
Nitric oxide, a simple molecule with immense biological importance, plays a critical role in various bodily functions. It’s primarily produced in the cardiovascular and nervous systems and acts as a neurotransmitter. The molecule gained significant attention after being named the “Molecule of the Year” in 1992 and has been the subject of over 140,000 studies, leading to numerous pharmaceutical developments like Viagra.
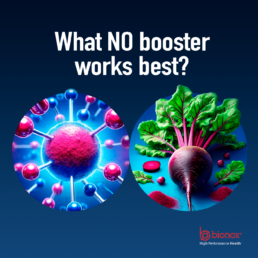
Arginine: The Traditional Nitric Oxide Booster
Arginine, a semi-essential amino acid, has long been the focal point in nitric oxide research. The body naturally produces Arginine, which is also found in various foods. It transforms into nitric oxide through an enzyme called nitric oxide synthase, present in the endothelium of arteries. However, the effectiveness of Arginine supplementation has been debated, especially concerning its conversion rate into nitric oxide in older individuals. Despite these discussions, Arginine’s role in boosting nitric oxide remains substantiated by extensive research and clinical practice.
Beets: The Emerging Nitric Oxide Source
Beets, and other nitrate-rich foods like arugula and spinach, represent an alternative pathway for nitric oxide production. Some people who have products to sell are saying that only beets rank among the best nitric oxide boosters.
The nitrates in these foods convert into nitric oxide through a process involving oral microbiome and stomach acid, independent of the nitric oxide synthase pathway. This feature makes them particularly beneficial for individuals with endothelial dysfunction. However, sourcing quality nitrate-rich supplements can be challenging, and factors like oral microbiome health and stomach acid levels significantly influence their effectiveness.
Comparing Arginine and Beets
When comparing arginine and beets, each has its advantages and drawbacks. Arginine’s benefits are well-researched, and it’s naturally produced by the body and it is well known as one of the best nitric oxide boosters around. However, it may not be as effective in older individuals due to decreased nitric oxide synthase activity. On the other hand, beets offer a nitric oxide synthase-independent pathway but rely heavily on the individual’s oral and gut health for conversion.
Practical Applications and Products
In clinical practice, understanding each patient’s unique health profile is crucial when choosing between Arginine and beets. For those with compromised nitric oxide synthase activity or seeking a natural alternative, beets and other nitrate-rich foods can be an excellent choice. Conversely, Arginine supplements, often combined with other co-factors and antioxidants, can offer a more controlled and research-backed approach.
Several products on the market cater to these needs, ranging from Arginine-based supplements to beetroot extracts and greens powders high in nitrates. Additionally, testing methods like saliva nitrate strips and digital pulse wave analyzers can help track nitric oxide levels and arterial health, guiding supplementation choices.
Poorly Designed Studies
The Vascular Interaction With Age in Myocardial Infarction (VINTAGE MI) clinical trial, a notable study on the use of L-arginine in acute myocardial infarction patients, has sparked considerable debate in the medical community. This study, published in the January 2006 issue of the Journal of the American Medical Association (JAMA), sought to determine if adding L-arginine to standard post-heart attack therapy could reduce vascular stiffness and improve heart function over a six-month period.
Study Design and Findings
- Context & Objective: Recognizing L-arginine as a substrate for nitric oxide synthase and its potential to reduce vascular stiffness, the study aimed to assess its efficacy in improving cardiac function post-heart attack.
- Methodology: It was a single-center, randomized, double-blind, placebo-controlled trial, enrolling 153 patients who had experienced a first ST-segment elevation myocardial infarction. The participants were randomized to receive either L-arginine (goal dose of 3 g three times a day) or a placebo for six months.
- Results: The study found no significant improvement in vascular stiffness or left ventricular ejection fraction in the L-arginine group compared to the placebo group. Notably, six participants (8.6%) in the L-arginine group died during the study, while no deaths were reported in the placebo group. This led to safety concerns and the early closure of the trial by the data and safety monitoring committee.
Criticisms and Controversies
The VINTAGE MI trial has been criticized for several reasons:
- Dosage and Administration: Some experts argue that the dosage of L-arginine used was inadequate for therapeutic effects. Furthermore, questions have been raised about the absorption and metabolism of L-arginine in the patients enrolled in the study.
- Patient Selection: Concerns have been raised regarding the specific cohort of patients chosen for the study, particularly their health status and how representative they were of the general population experiencing heart attacks.
- Study Design: Critics have pointed out potential flaws in the study’s design, including the single-center nature of the trial and the methods used to measure outcomes such as vascular stiffness and heart function.
Implications for Clinical Practice
While the VINTAGE MI trial suggests potential risks associated with L-arginine supplementation post-heart attack, it is important to consider these results within the broader context of L-arginine research and cardiovascular health. The role of L-arginine in cardiovascular therapy, particularly in non-post-infarction contexts, continues to be supported by a body of research indicating its benefits in vascular health and nitric oxide production.
The VINTAGE MI trial represents a critical piece of research in the ongoing evaluation of L-arginine’s role in heart health. However, its findings should be interpreted with caution and in conjunction with other studies. As with any supplement or medication, the suitability of L-arginine for individual patients should be assessed based on a comprehensive understanding of their overall health, the specific health condition being treated, and the broader scientific evidence available.
In the debate between Arginine and beets for nitric oxide boosting, there’s no one-size-fits-all answer. Each has its unique benefits and limitations. The choice largely depends on individual health factors, goals, and preferences. Doing a good heavy metal detox is going to be very helpful in any case, no matter what you take.
By understanding the nuances of these nitric oxide boosters, one can make informed decisions to optimize health and wellness.
If you would like in depth information watch my video presentation from ANMA below.
Heavy Metals and Nitric Oxide Synthase
Introduction
Heavy metals, such as lead, mercury, cadmium, and arsenic, are environmental pollutants that can accumulate in the body and pose significant health risks. One of the lesser-known consequences of heavy metal exposure is the negative impact on nitric oxide synthase (NOS) production. NOS is an enzyme responsible for producing nitric oxide (NO), a signaling molecule that plays a crucial role in various physiological processes, including vasodilation, immune response, and neurotransmission. This article will discuss the mechanisms through which heavy metals can decrease NOS production, the health implications of this reduction, and strategies to counteract these effects, with references to scientific studies supporting these claims.
Mechanisms of Heavy Metal-Induced NOS Inhibition
A. Oxidative Stress
Heavy metals can induce oxidative stress, which is characterized by an imbalance between the production of reactive oxygen species (ROS) and the body’s antioxidant defense mechanisms (1). Excessive ROS production can lead to the inactivation of NOS and a decrease in NO production (2). Oxidative stress also contributes to the uncoupling of endothelial NOS (eNOS), a process in which the enzyme produces superoxide instead of NO, further exacerbating the negative effects on NOS activity (3).
B. Disruption of NOS Expression and Function
Heavy metals can directly interact with NOS enzymes or alter their expression, decreasing NO production (4). For example, cadmium has been shown to inhibit NOS activity by displacing essential cofactors, such as zinc, which are necessary for proper enzyme function (5). Additionally, heavy metals can interfere with the cellular signaling pathways that regulate NOS expression, ultimately suppressing enzyme production (6).
C. Inhibition of NO Bioavailability
Heavy metals can also decrease NO bioavailability by increasing the production of molecules that scavenge and inactivate NO, such as asymmetric dimethylarginine (ADMA) (7). ADMA, an endogenous inhibitor of NOS, competes with L-arginine, the substrate for NOS, for binding to the enzyme, thereby decreasing NO production (8).
Health Implications of Heavy Metal-Induced NOS Inhibition
A. Cardiovascular Disease
Decreased NOS activity and NO production from heavy metal exposure can impair endothelial function, reducing vasodilation and increasing blood pressure (9). This can contribute to the development of cardiovascular diseases, such as atherosclerosis and hypertension (10).
B. Neurological Disorders
NO is essential for normal neurotransmission and brain function. Reduced NOS activity and NO production due to heavy metal exposure can lead to altered neurotransmitter release, synaptic plasticity, and neuronal survival, contributing to the development of neurological disorders such as Parkinson’s disease and cognitive impairment (11, 12).
C. Impaired Immune Response
NO plays a critical role in the immune response by modulating the function of immune cells and influencing cytokine production. Reduced NO production due to heavy metal-induced NOS inhibition can impair the immune system’s ability to fight off infections and maintain proper inflammatory responses (13).
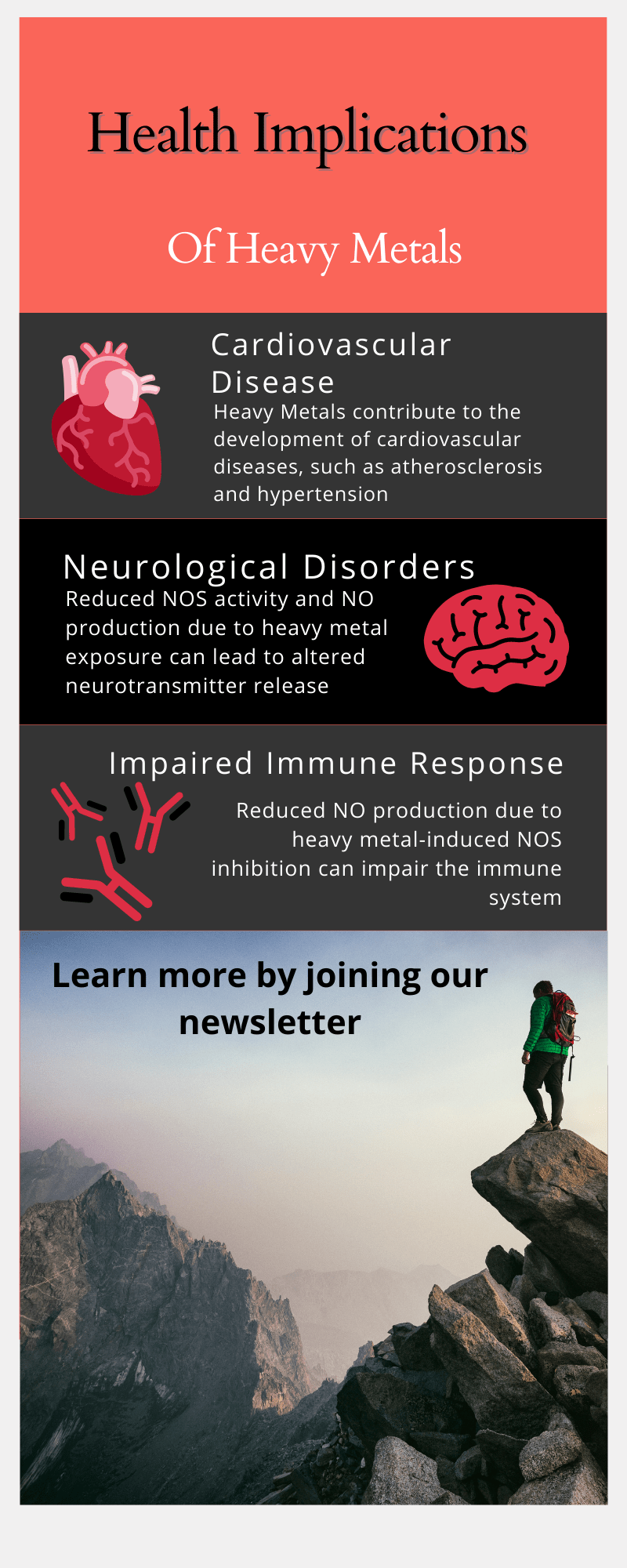
Strategies to Counteract Heavy Metal-Induced NOS Inhibition
A. Chelation Therapy
Chelation therapy involves the administration of chelating agents, such as ethylenediaminetetraacetic acid (EDTA) or dimercaptosuccinic acid (DMSA), which bind to heavy metals and facilitate their excretion from the body. By reducing the body’s burden of heavy metals, chelation therapy can help restore NOS activity and improve overall health (14).
B. Antioxidant Supplementation
Antioxidants, such as vitamins C and E, can help counteract oxidative stress from heavy metals and protect NOS activity (15). Supplementation with antioxidants may help restore NO production and support overall health in individuals exposed to heavy metals.
C. Nutritional and Lifestyle Interventions
Consuming a diet rich in antioxidants, essential nutrients, and anti-inflammatory compounds can help support NOS activity and counteract the effects of heavy metal exposure (16). Additionally, engaging in regular physical activity, maintaining healthy body weight, and avoiding exposure to environmental pollutants can further protect NOS function and overall health.
Conclusion
Heavy metals can negatively impact nitric oxide synthase production through various mechanisms, including inducing oxidative stress, disrupting NOS expression and function, and inhibiting NO bioavailability. The detrimental effects of heavy metals on NOS activity can contribute to the development of cardiovascular diseases, neurological disorders, and impaired immune responses. Chelation therapy, antioxidant supplementation, and nutritional and lifestyle interventions can be employed to counteract these effects. Individuals can proactively protect their health and mitigate the risks associated with heavy metal exposure by understanding the relationship between heavy metals and NOS production.
A Comprehensive Approach to Support NOS Production
This article discusses how can some components contribute to heavy metal detoxification and supports NOS production.
EDTA
Ethylenediaminetetraacetic acid (EDTA) is a well-known chelating agent that binds to heavy metals, such as lead, cadmium, and mercury, facilitating their excretion from the body (17). By removing heavy metals, EDTA can help restore NOS activity and mitigate the negative effects of these metals on nitric oxide (NO) production (18).
Modified Citrus Pectin
Modified citrus pectin is a form of pectin that has been altered to improve its bioavailability and absorption. It has been shown to bind and remove heavy metals from the body, such as lead, mercury, and cadmium (19). Modified citrus pectin can help protect NOS activity and support NO production by aiding in heavy metal detoxification.
Chlorella
Chlorella is a single-celled green alga that has been shown to possess heavy metal-binding properties, particularly for mercury (20). By assisting in removing heavy metals from the body, chlorella can help alleviate the negative effects of these metals on NOS production and support overall health.
Cilantro
Cilantro, also known as coriander, has been shown to have heavy metal-chelating properties, particularly for lead and mercury (21). By aiding in detoxifying heavy metals, cilantro can help protect NOS activity and support NO production.
Shilajit
Shilajit, a natural resinous substance found in the Himalayas, has been reported to have antioxidant and anti-inflammatory properties, which may help counteract heavy metal-induced oxidative stress and inflammation (22). Shilajit can help protect NOS activity and maintain NO production by reducing oxidative stress. Additionally, shilajit has been reported to possess metal-chelating properties, which may further contribute to its heavy metal detoxification effects (23).
Zeolite
Zeolites are natural or synthetic minerals with a unique porous structure, which allows them to bind to and trap heavy metals, such as lead, cadmium, and mercury (24). By assisting in removing heavy metals from the body, zeolites can help protect NOS activity and support NO production.
References:
(1) Valko, M., Morris, H., & Cronin, M. T. (2005). Metals, toxicity and oxidative stress. Current Medicinal Chemistry, 12(10), 1161-1208. https://doi.org/10.2174/0929867053764635
(2) Förstermann, U., & Sessa, W. C. (2012). Nitric oxide synthases: regulation and function. European Heart Journal, 33(7), 829-837. https://doi.org/10.1093/eurheartj/ehr304
(3) Förstermann, U., & Münzel, T. (2006). Endothelial nitric oxide synthase in vascular disease: from marvel to menace. Circulation, 113(13), 1708-1714. https://doi.org/10.1161/CIRCULATIONAHA.105.602532
(4) Brüne, B., Schmidt, K. U., & Ullrich, V. (1990). Activation of soluble guanylate cyclase by carbon monoxide and inhibition by superoxide anion. European Journal of Biochemistry, 192(2), 683-688. https://doi.org/10.1111/j.1432-1033.1990.tb19283.x
(5) Ercal, N., Gurer-Orhan, H., & Aykin-Burns, N. (2001). Toxic metals and oxidative stress part I: mechanisms involved in metal-induced oxidative damage. Current Topics in Medicinal Chemistry, 1(6), 529-539. https://doi.org/10.2174/1568026013394831
(6) Pacher, P., Beckman, J. S., & Liaudet, L. (2007). Nitric oxide and peroxynitrite in health and disease. Physiological Reviews, 87(1), 315-424. https://doi.org/10.1152/physrev.00029.2006
(7) Kielstein, J. T., & Cooke, J. P. (2005). Cardiology and nephrology converge on a common problem: asymmetric dimethylarginine (ADMA), an endogenous inhibitor of nitric oxide synthase, predicts cardiovascular events. Journal of the American Society of Nephrology, 16(9), 2454-2457. https://doi.org/10.1681/ASN.2005060610
(8) Böger, R. H. (2006). Asymmetric dimethylarginine, an endogenous inhibitor of nitric oxide synthase, explains the “L-arginine paradox” and acts as a novel cardiovascular risk factor. Journal of Nutrition, 136(10), 2882S-2887S. https://doi.org/10.1093/jn/136.10.2882S
(9) Vaziri, N. D. (2008). Mechanisms of lead-induced hypertension and cardiovascular disease. American Journal of Physiology-Heart and Circulatory Physiology, 295(2), H454-H465. https://doi.org/10.1152/ajpheart.00158.2008
(10) Navas-Acien, A., Guallar, E., Silbergeld, E. K., & Rothenberg, S. J. (2007). Lead exposure and cardiovascular disease: a systematic review. Environmental Health Perspectives, 115(3), 472-482. https://doi.org/10.1289/ehp.9785
(11) Farina, M., Avila, D. S., da Rocha, J. B., & Aschner, M. (2013). Metals, oxidative stress and neurodegeneration: a focus on iron, manganese and mercury. Neurochemistry International, 62(5), 575-594. https://doi.org/10.1016/j.neuint.2012.12.006
(12) Sanders, T., Liu, Y., Buchner, V., & Tchounwou, P. B. (2009). Neurotoxic effects and biomarkers of lead exposure: a review. Reviews on Environmental Health, 24(1), 15-45. https://doi.org/10.151 5/reveh.2009.24.1.15
(13) Bogdan, C. (2001). Nitric oxide and the immune response. Nature Immunology, 2(10), 907-916. https://doi.org/10.1038/ni1001-907
(14) Flora, S. J., & Pachauri, V. (2010). Chelation in metal intoxication. International Journal of Environmental Research and Public Health, 7(7), 2745-2788. https://doi.org/10.3390/ijerph7072745
(15) Lobo, V., Patil, A., Phatak, A., & Chandra, N. (2010). Free radicals, antioxidants and functional foods: Impact on human health. Pharmacognosy Reviews, 4(8), 118-126. https://doi.org/10.4103/0973-7847.70902
(16) Crinnion, W. J. (2010). The role of nutritional supplements in the treatment of heavy metal toxicity. Alternative Medicine Review, 15(1), 33-47. http://archive.foundationalmedicinereview.com/publications/15/1/33.pdf
(17) Flora, S. J., & Pachauri, V. (2010). Chelation in metal intoxication. International Journal of Environmental Research and Public Health, 7(7), 2745-2788. https://doi.org/10.3390/ijerph7072745
(18) Vaziri, N. D. (2008). Mechanisms of lead-induced hypertension and cardiovascular disease. American Journal of Physiology-Heart and Circulatory Physiology, 295(2), H454-H465. https://doi.org/10.1152/ajpheart.00158.2008
(19) Eliaz, I., Weil, E., & Wilk, B. (2019). Integrative medicine and the role of modified citrus pectin/alginates in heavy metal chelation and detoxification – five case reports. Functional Foods in Health and Disease, 8(12), 430-443. https://doi.org/10.31989/ffhd.v8i12.569
(20) Uchikawa, T., Yasutake, A., Kumamoto, Y., Maruyama, I., Kumamoto, S., & Ando, Y. (2011). The influence of Parachlorella beyerinckii CK-5 on the absorption and excretion of methylmercury (MeHg) in mice. Journal of Toxicological Sciences, 36(1), 121-130. https://doi.org/10.2131/jts.36.121
(21) Aga, M., Iwaki, K., Ueda, Y., Ushio, S., Masaki, N., Fukuda, S., … & Ito, Y. (2001). Preventive effect of Coriandrum sativum (Chinese parsley) on localized lead deposition in ICR mice. Journal of Ethnopharmacology, 77(2-3), 203-208. https://doi.org/10.1016/S0378-8741(01)00289-X
(22) Carrasco-Gallardo, C., Guzmán, L., & Maccioni, R. B. (2012). Shilajit: a natural phytocomplex with potential procognitive activity. International Journal of Alzheimer’s Disease, 2012, 674142. https://doi.org/10.1155/2012/674142
(23) Bhattacharyya, S., & Pal, D. (2013). In vitro study of the effects of Shilajit on the activities of Ehrlich ascites tumor cells. Pharmaceutical Biology, 51(2), 269-272. https://doi.org/10.3109/13880209.2012.727360
(24) Selvam, T., Schwieger, W., & Dathe, W. (2017). The potential of natural and modified zeolites for heavy metal capture in contaminated waters. In Natural Mineral Nanotubes (pp. 363-380). CRC Press. https://doi.org/10.1201/b18522-16